Introduction
Novel carbon dioxide removal (CDR) technologies face the challenge of not fitting into the established carbon management framework of the historic voluntary carbon market. This is particularly true for open system technologies, such as Enhanced Weathering (EW). EW represents a convergence of natural sciences with carbon management and accounting, which, separately, have complex and highly defined working languages. In the emerging CDR industry, there is a lack of widespread familiarity with EW technology components, creating confusion about how to handle it within traditional certification and carbon management frameworks. Equally, natural scientists are not familiar with carbon management terminology. As such, we see the need to clarify definitions that have implications for understanding the roles and responsibilities of carbon management.
The terminology below is defined with specific reference to EW in agricultural settings. We so far do not include more widely applicable terminology such as ‘additionality’ and do not impose recommendations on how to operationally treat or measure factors (e.g., counterfactuals); we only define them. The document will be updated as required to be consistent with emerging consensus, standards, and the best available science.
The stages of EW CDR are illustrated in Fig. 1, which further clarifies the relationship between stages, processes, and credit issuance. The relationship of CO2 loss processes (including downstream CO2 losses and reversals) relative to credit issuance is also shown. Note that there is overlap between stages, reflecting the open system nature of the process.
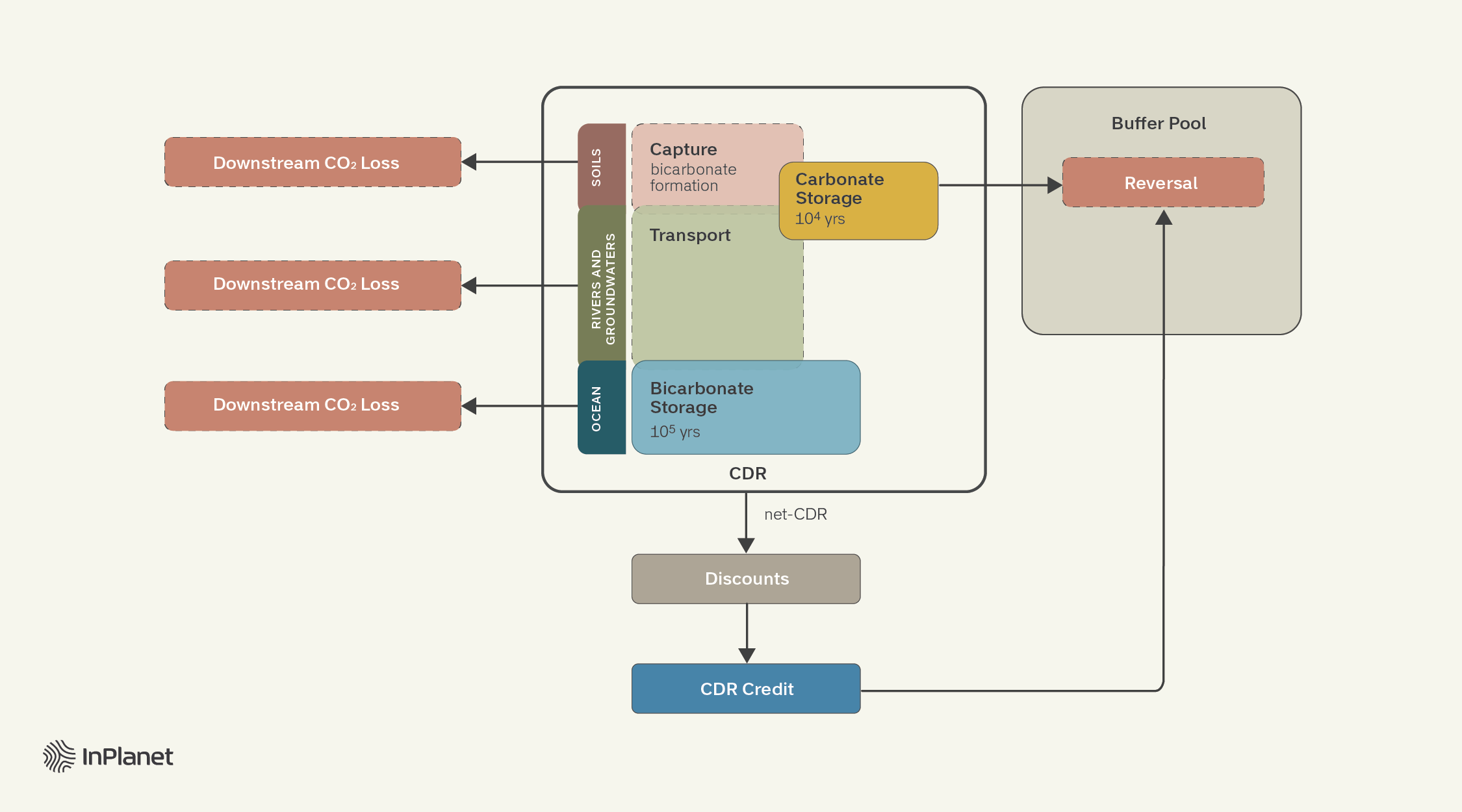
Definitions
Carbon Dioxide Removal (CDR)
Following the IPCC (2022) recommendation, CDR is defined as “Human activities capturing CO2 from the atmosphere and storing it durably in geological, land or ocean reservoirs, or in products. This includes human enhancement of natural removal processes, but excludes natural uptake not caused directly by human activities.”
CDR encompasses the capture process, transfer through different carbon pools, and final storage within a durable pool within a non-atmospheric reservoir (Smith et al., 2023).
Capture
The point where atmospheric CO2 that is dissolved in water is transformed to bicarbonate (Smith et al., 2023). Capture can occur in close proximity to the rock powder application at the weathering site, within the agricultural soil setting. Capture can also occur during transport, illustrated by the stage overlap in Fig. 1. Capture is often referred to as ‘removal’ but should not be confused with the entire CDR process, which involves both capture and storage (see above).
Transport
Transport broadly refers to the stage of CDR after the point of capture and prior to storage; however, the two cannot be fully separated, as shown in Fig. 1. Transport includes the transfer of bicarbonate as an aqueous phase through the soil system and terrestrial water networks (groundwaters and rivers) and exit into the ocean. Transport involves physical-chemical processes, including interactions with soil components (e.g., fluids, minerals, organic matter) and reactions that occur within rivers or estuaries (degassing, carbonate precipitation, clay formation). Some of these processes result in downstream CO2 losses.
Storage
Storage marks the addition of CO2 that has been removed from the atmosphere to a durable reservoir. Long-term storage of carbon occurs primarily as bicarbonate, usually in the ocean or in groundwaters, or as carbonate minerals in soils (Campbell et al., 2022; Smith et al., 2023). Thus, there are two primary durable pools for EW activities, representing two theoretically distinct CDR pathways. The relative importance of each removal pathway and final storage will vary with feedstock choice, climate, soil type, land management practices, and river chemistry. In nature, both pathways can exist in parallel, but carbonate storage will likely be the minority on a global scale.
In the case that storage occurs as bicarbonate in the ocean reservoir, ‘storage’ marks a time point after transportation out of the soil system through groundwater, rivers, and estuaries to the ocean reservoir. This could be months to years after capture when EW is applied to an agricultural setting. Bicarbonate is considered stable in the ocean on the order of tens-to-hundreds of thousands of years (Goodwin et al., 2007; Kanzaki et al., 2023; Renforth and Henderson, 2017; Smith et al., 2023) exceeding all definitions of permanence used in carbon management practices and by standard or regulatory bodies. Some bicarbonate may be stored in long-term groundwater.
The second pathway, often referred to as mineralization, is where carbon is stored as soil inorganic carbon (pedogenic carbonate minerals). Any carbonate minerals formed in soils are also considered permanent carbon removal (Campbell et al., 2022) despite the potential for shorter-term re-mobilization. Carbonates precipitated downstream of the removal site could also be considered storage, but accounting for this fraction is more difficult. CDR from carbonate precipitation is less effective than bicarbonate storage because CO2 is re-released during carbonate mineral precipitation. Any carbonate precipitation, therefore, represents a downstream CO2 loss.
Downstream CO2 loss
Post-capture loss of CO2 resulting in a decrease in net-CDR estimates, equivalent to a decrease in CDR efficiency, typically related to physical-chemical processes. We recommend avoiding the term ‘leakage’ in the context of physical-chemical CO2 losses to avoid confusion with ‘socio-economic leakage’. Distinction of the terms is preferred rather than relying on contextual information to identify leakage type.
Net-CDR
Net-CDR refers to a calculated estimate of the amount of carbon stored by CDR, representing the final mass of CO2 removed that can be commoditized into a carbon removal credit. Net-CDR estimates to account for CO2 loss due to, for example, operational emissions, any downstream CO2 loss that occurs during transport, and socio-economic leakage. Net-CDR estimates can be subject to discounting before final credit issuance to account for uncertainty.
Socio-economic leakage
We specify the term socio-economic leakage as the displacement of CO2 emissions by the project that drives a change in emissions outside of the project boundaries (Puro.earth, 2022; Wilcox et al., 2021). This is typically related to land use changes or alternative product development to satisfy market demand. For EW, examples of socio-economic leakage include i) the expansion of agricultural land in the event of a yield decrease, although there is no current scientific evidence for such a complication, and ii) the displacement of mining operations as rock powder demand increases.
Socio-economic leakage is often referred to as ‘leakage’ in some carbon management practices. However, we recommend avoiding the shortened term due to confusion with physical-chemical processes that result in downstream CO2 losses after removal, such as evasion in rivers. Distinction of the terms is preferred rather than relying on contextual information to identify leakage type.
System-wide uncertainties
Encompasses inherent uncertainties within CDR calculations based on both measurement uncertainty (instrumental external reproducibility) and the approach used, including the ability to resolve weathering signals due to physical-chemical, spatial or temporal effects. Uncertainties could further include those related to the quantification of downstream CO2 losses.
Durability
The physical-chemical integrity of carbon storage over time. Applies to post-credit issuance.
Permanence
Refers to the time over which carbon is durably stored.
Reversals
Reversals are defined as storage failure or the premature release of stored CO2 (Microsoft and Carbon Direct, 2023), that directly affect durability. We specify that reversals are a natural or anthropogenic process resulting in the failure of carbon storage that is external to the EW operation. As the definition specifies a failure of storage, reversals impact the integrity of certified carbon removal credits. Reversals require tools for liability and insurance against storage failure (such as a buffer pool). For EW, storage failure is only plausible for carbonate storage as it is subjected to future land use changes (e.g. fertilizer use). Loss of CO2 from ocean bicarbonate storage is treated as a downstream CO2 loss.
Discounts
Discounts are systematically applied to net CDR estimates to account for system-wide uncertainties (Frontier). This includes the ability to accurately quantify downstream CO2 losses.
Buffer pool
A buffer pool is an unsold allotment of carbon removal credits that can be used to cover the liability of reversals.
Baseline scenario
A baseline scenario is defined by the absence of the EW operation, comparable to a scientific control in the EW project activity.
Counterfactual
Counterfactuals represent the CDR estimate from the baseline scenario. The counterfactual must be subtracted from the gross CDR estimate of the EW activity as part of the net-CDR calculation.
References
Campbell, J.S., Foteinis, S., Furey, V., Hawrot, O., Pike, D., Aeschlimann, S., Maesano, C.N., Reginato, P.L., Goodwin, D.R., Looger, L.L., Boyden, E.S., Renforth, P., 2022. Geochemical Negative Emissions Technologies: Part I. Review. Front. Clim. 4.
Goodwin, P., Williams, R.G., Follows, M.J., Dutkiewicz, S., 2007. Ocean-atmosphere partitioning of anthropogenic carbon dioxide on centennial timescales. Glob. Biogeochem. Cycles 21. https://doi.org/10.1029/2006GB002810
Kanzaki, Y., Planavsky, N.J., Reinhard, C.T., 2023. New estimates of the storage permanence and ocean co-benefits of enhanced rock weathering. PNAS Nexus 2, pgad059. https://doi.org/10.1093/pnasnexus/pgad059
Microsoft, Carbon Direct, 2023. Criteria for High-Quality Carbon Dioxide Removal.
Puro.earth, 2022. Enhanced Rock Weathering Methodology.
Renforth, P., Henderson, G., 2017. Assessing ocean alkalinity for carbon sequestration. Rev. Geophys. 55, 636–674.
Smith, S.M., Geden, O., Nemet, G.F., Gidden, M.J., Lamb, W.F., Powis, C., Bellamy, R., Callaghan, M.W., Cowie, A., Cox, E., Fuss, S., Gasser, T., Grassi, G., Greene, J., Lück, S., Mohan, A., Müller-Hansen, F., Peters, G.P., Pratama, Y., Repke, T., Riahi, K., Schenuit, F., Steinhauser, J., Strefler, J., Valenzuela, J.M., Minx, J.C., 2023. The State of Carbon Dioxide Removal – 1st Edition. The State of Carbon Dioxide Removal. https://doi.org/10.17605/OSF.IO/W3B4Z
Wilcox, J., Kolosz, Ben, Freeman, Jeremy, 2021. CDR Primer.
Intergovernmental Panel On Climate Change (IPCC). Annex I: Glossary. in Climate Change 2022 – Mitigation of Climate Change 1793–1820 (Cambridge University Press, 2023). doi:10.1017/9781009157926.020.
Authored by:
Roos de Bruijn
Business Development
Dr. Matthew Clarkson
Head of Carbon
Dr. Christina Larkin
Head of Science & Research